What’s all this?
In 10 seconds,
- A high performance, 7MHz, 5 watt SSB rig
- Draws just 24 mA of current
- 90 dB dynamic range, 80 dB close-in dynamic range
- 3D printed rock stable, slow tuning PTO with zero backlash.
- 3D printed toroids
- You have all the parts in the junk box or you can buy them tonight at the vendor night.
- Very stable, slow tuning VFO with no backlash
- Costs less than 20 dollars to build, even in new parts.
The Circuit Diagram
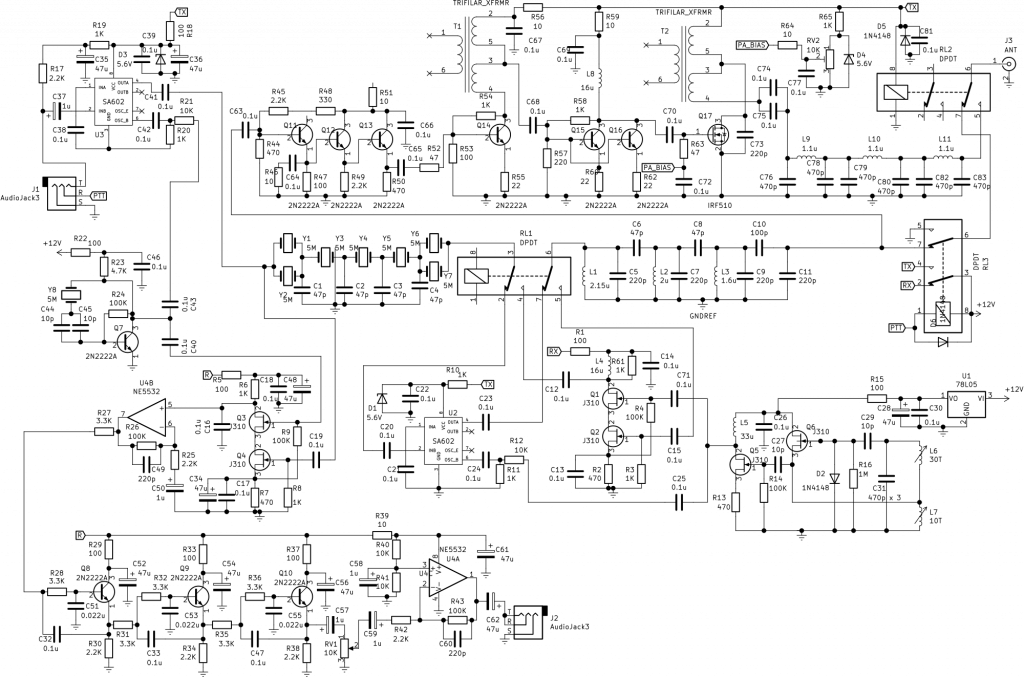
Full Circuit Diagram
Download the circuit diagram in PDF |
Download 3 D print files of the PTO
Isn’t analog radio an anachronism? Are we just being nostalgic? What’s wrong with the Si5351?
Last year, when we had to isolate ourselves for a week, I managed to grab some components, an Antuino (the RF lab in the box), some basic tools and set about making a slightly souped up version of Roy Lewallen’s Optimized Transceiver from our room. The experience of a well designed analog rig was truly enlightening. There were no spurs, the audio was crystal clear and with presence.
When David NM0S and I were discussing this year’s FDIM, I suggested that we do a return to analog radios and he jumped at it! So here we are, talking analog radios in 2022.
Here is the memo : The analog never died. The world is analog all the way, until you descend into Quantum madness. The antennas are analog, Maxwell died a content, analog man. Our radios, ultimately, are analog machines and we are all analog beasts too. Amateur Radio technology has evolved into the digital domain. However, it has only made it easier for us to do analog with computers to simulate and print our circuits.
So, it’s time to bid good bye to our Arduinos and Raspberry Pis and build an Analog Radio for ourselves. So let’s see what we can achieve in hindsight, a return to our native land and a rethink of our approaches.
The radio is called Daylight Again, a nod to being back at the FDIM in 2022 after a gap of two years. It is named after the Crosby, Stills, Nash and Young’s song that had been humming all the time while put this radio together, emerging after 2 years of lockdown. This radio that took two days to come together, no actually two years! That’s: parts of it got built and stowed away, thoughts were struck in the shower, questions popped up during early morning cycle rides and notes and circuits were scribbled in the notebook.
I must take the first of many diversion here: I hope you all maintain a notebook. Write down the date and whatever you thought or did on the bench and the result. Nothing is trivial enough to leave out. Wisdom comes to those who write notes.
I started to build this on Saturday the 14th May and I checked into the local SSB net on Monday morning, the 16th May 2022.
Back to the radio. What can an analog radio do that will appeal to us homebrewers?
-
- Simple to build (?) This is a myth as this paper will demonstrate. It may be easy to duplicate a design from the QRP Journal or the Sprat, but to design a radio that is stable, sensitive, selective and sounds sweet on air is not trivial. It needs thinking and revisiting our assumptions and habits (like the diode mixers).
- Low power: The radio draws just 24 mAYes, an analog radio can be really really lean. Low power is not just a show-off. It has some real purpose. One can work for weeks from a single pack of AA cells. Working your rig off a battery pack eliminates a large species of noise and spurs; As things stay cool inside, the VFO drift is much lower and one could carry the rig on a cycle!
- Low noise VFO.What’s the big deal here? All local oscillators are the same, isn’t it? Not really. Consider each VFO to be an AM transmitter that splatters around the carrier. As the VFO splatter mixes with an incoming signal that is strong and yet off your receiver frequency, to the listener it appears as if the signal has splatter. Actually, the signal would be entirely clean, it is your own local oscillator’s splatter that you are hearing.
- Having clean VFO is the most important way of increasing the dynamic range of your radio. A free running JEFT VFO that has sufficient power and a good Q components, will be unmatched by any synthesized or direct sampling radios. The math is all on the side of the free running VFO. We are talking -150 db/Hz at 10 KHz spacing, by comparison the Si5351 is -125 db/Hz, it is 300 times worse.
- Stability of 2 Hz/15 second. Can it decode FT8? Actually yes, we have had regular decodes after 15 minutes of warm up. It stays stable enough for CW and SSB. WSJT-X suite is the main challenge. We need a stability of better than 2 Hz/15 second period for the WSJT-X suit to work. We do this by keeping the operating frequency of the VFO low, eliminating heat around the VFO and using sturdy but ugly, short and stiff point to point wiring.
- Dynamic Range of 90 dB and close-in range of 80 dB. The real, tough, beef eating hams don’t care for dynamic range, we operate! Right? Wrong. The bands have changed. Signals tend to cluster within narrow pockets. All the QRPers hang out on 7040, the PSK gang is over at 7060, the FT8 kiddies are parked at 7074. The local charminar net at 7080, long after it is closed, has the hanger-ons late into the noon. We need radios where we can clearly make out a CW pileup where you can hear 20 different stations at once.The 90 dB is actually a very good number. The gains beyond this are marginal. Unless you are operating with full power stations within close neighbourhood on the same band as you, 80 dB of close-in dynamic range is enough to win you CW sweepstakes. Ask Rob Sherwood (https://www.youtube.com/watch?v=GNh0wP9PlsM).
- Why SSB? Just because it is a more complex thing to build. It is easier to add CW to an SSB rig rather than add SSB to a CW rig. The amplifiers need to be linear, sideband needs to be suppressed etc. We may add CW to this rig later.
- Undo the Lore.We homebrewers tend to stick to the beaten path. We build exactly the same radios over and over again. When was the last time you didn’t use a diode mixer? Or build radios that didn’t use 50 ohms interconnect? It is about time.
I must warn you there is mathematics ahead. Not too much, but enough to keep you on your toes.
The Daylight radio has a few big ideas that are interesting enough to be tried out. I am leaving out the bad ideas (like varactor tuning and using class E amplification) from the scope of this paper.
The Cascode Mixer
Remember the 70s when Doug, W1FB built those radios with 40673 dual-gate MOSFETs? Well, they are back! It is 2022 and they are exhibiting good dynamic range, we are rebooting the old heros.
The dual-gate MOSFETs are, surprisingly enough, still being manufactured. Mouser lists tens of thousands of them, they are cheap but available only as SMD parts. However, you can build a virtual dual-gate FET from two JFETs, it works all the same. A bag of 100 J310s is still sold for a few dollars at Dan’s (. With careful design, a cascode can provide us with +8dbm of input intercept and a gain of 10 db. This might not seem much to most but it is.
This is more than respectable, it is downright awesome. Here is why: The cascode mixer with +8 dBm of Input intercept(IIP3) and with 10 dB gain over it has an OIP3 (output intercept) a huge +18 dbm (calculated as IIP3 + gain).
In comparison, the diode mixer with +15dbm input intercept has a loss of 7 db and noise figure of 7db (actually the loss is the noise figure). Thus a diode mixer’s output intercept is +8 dBm (again calculated as IIP3 + gain, that is negative loss in this case) as compared to +18 dBm of the JFET cascode.
There are several striking things about this mixer:
-
-
- Take very little VFO power The oscillator injection on Q1 is to the gate, it takes zero power of local oscillator injection (the current never flows into the JFET gate). This results in the VFO running cooler and with less drift and less current consumption!
- Needs no post-mix amplifier This mixer is insensitive to termination and it is unidirectional. The signals will not flow back from the drain to the source. You don’t need to follow this up with a post mixer amplifier that will draw even higher current. Conventionally, a diode mixer needs 5 milliwatts of local oscillator, which means that the oscillator itself must draw about 100 milliwatts to produce it and the diode mixers invariably need a post mix amplifier with about 20 ma of standing current, which, at 12 v will amount to 12 x0.02 = 240 milliwatts of power. In effect, together, the diode mixer and its post-mixer amplifier up the receive budget by 340 milliwatts. People of the GQRP and QRPARCI clubs have attempted DXCC with those power levels!
- You can pick your own working impedance. To get upto 10 dB of gain, you will need to set the input and the output impedances to around 2000 ohms. However, at lower the impedance and the gain will decrease but the other mixer parameters will remain as they are! You can put this into LTSpice and smoke it. This makes the job of matching input to an RF band pass filter or the output to a crystal filter very easy.
- The output has an unsuppressed local oscillator. There is no balance, at least, in this simple design. We can add balance by using another similar mixer in push-pull configuration though as a receiver front-end mixer, our purpose is served without needing to suppress the local oscillator. The RF front-end can directly drive the crystal filter. However, the cascode mixer is unsuitable as a transmit mixer due to this oscillator leakage and we will use an NE612 for that as discussed later.
- Noise figure is 4 dB. This is really sensitive. You can build high grade VHF/UHF mixers with this sensitivity.
- Input Intercept of +8 dBm This needs some illustration. +8 dBm is about 7 milliwatts of power. When you overload the mixer with an unwanted signal of 7 milliwatts, the distortion produces unwanted signals that appear to be 7 milliwatts too, it is where the distortion is equal to the signal itself. This is a theoretical limit.
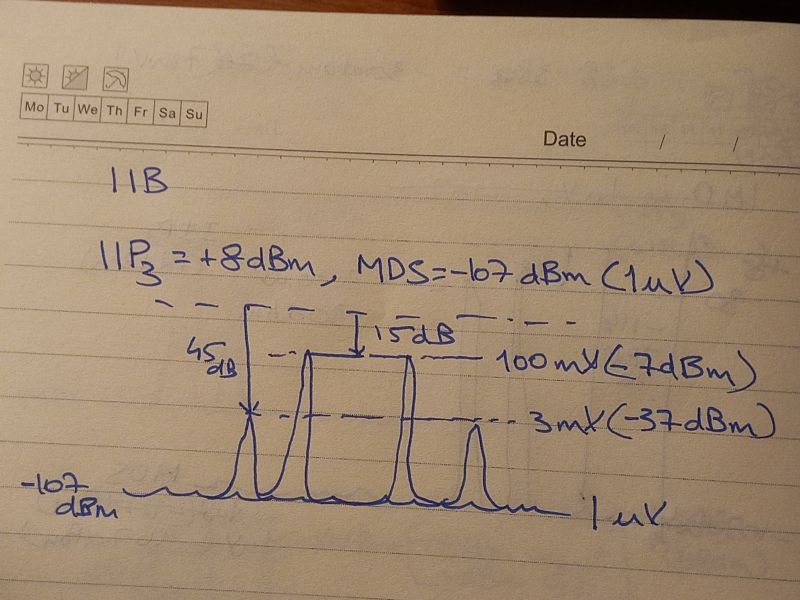
Notbook calculations of the IMD
As you start backing off the overloading signal, for each db drop in the signal’s strength, the distortion drops by 3 dB. So, let’s imagine that we now drive the mixer with -7 dBm signal, the output, which is below 15 dB below the IIP3, accordingly the distortion would drop to by 45 dB(15×3), now the distortion signals appear as (8 dBm- 45 dB) -37dBm signal.
Let me pick a more interesting RF signal of 7,000 uv, or -30 dBm. This is 38 db below the intercept level, accordingly, the IMD will be down by 38×3 = 114dB below the IIP3. The distortion is now at -106 dBm (1uV). Now, the distortion has gone into the noise floor. You can no longer hear the distortion. This is the dynamic range of the receiver. Impressive.
Controlled Gain, Block by Block
Our craft consists of stringing together filters, amplifiers, mixers and oscillators together.
One wonders : Is there a point in putting an IF amplifier at all? Should I add an RF amplifier? Is it better to replace the diode mixer with an NE612? It is confusing all the way!
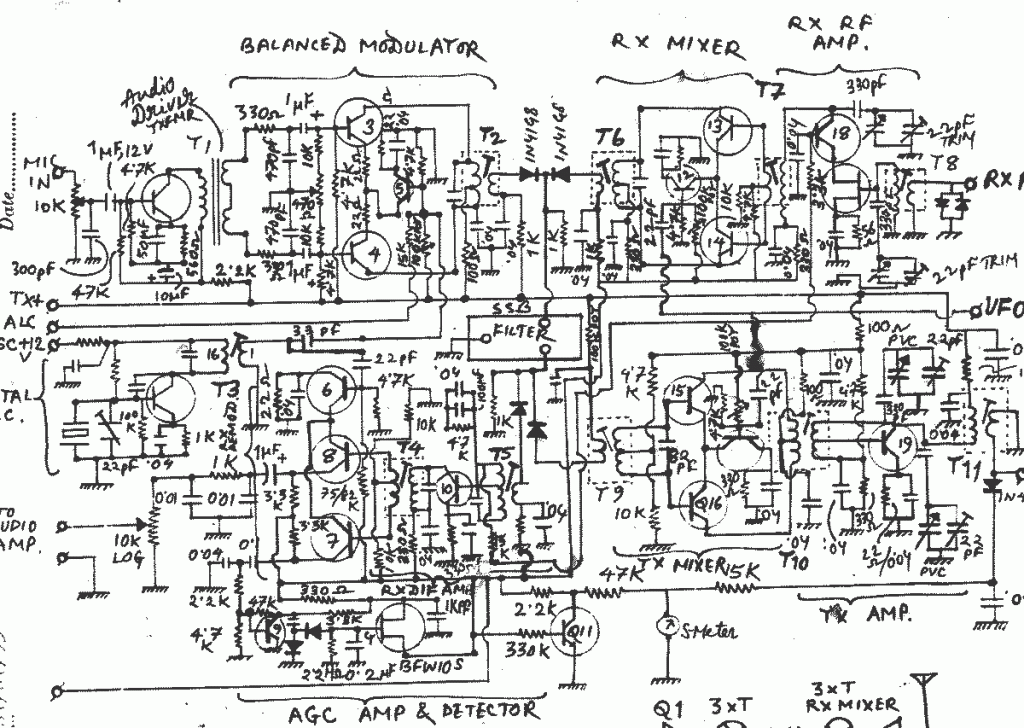
NR60, a homebrew design of the 1980s that wasn’t optimized for distortion
One assumed that more gain in a receiver meant better sensitivity. If the receiver sensitivity was improved, would it become much better? Actually, the converse is true. If the receiver is sensitive enough to be able to hear the atmospheric noise, then more gain will only overload the receiver.
How does one really figure out which stage is overloading? How much gain is optimal in each stage? How do we identify the offending weak stage in a radio’s line up of blocks? Some receivers turn out to be very sweet and clear even though they are simple while other simple ones don’t work just as well.
The art of building radios seems to be wrapped in some kind of black magic.
Well, it is no black magic, it is simple mathematics. You can accurately predict how sensitive a receiver will be (by calculating the minimum discernable signal), how clear the transmission will be (by predicting the IMD : Intermodulation Distortion), how loud the audio will be etc., using a simple online calculator. This tool was first written in the 70s by Wes Hayward, W7ZOI which was subsequently published on the floppy disk that came with his book – Introduction to Radio Frequency Design.
Diversion: This book is a classic, now available as a PDF. I would urge you to get it and take a print out and read it slowly, one page at a time. It is a far better investment than flipping through rigpix.com searching for your Radio porn.
It works simply by filling in a table of columns, each column represents one stage of either a transmitter or a receiver. For each stage you specify the noise figure, gain and output intercept (OIP3). Most RF chips, or circuit blocks do specify their performance in these three critical parameters.
[A Tip: In passive circuit blocks like the filters or diode mixers, the noise figure is the same as the loss. So, if a bandpass filter has a loss of 2dB, consider that to be its noise figure as well. For passive filters you can assume the OIP3 intercept to be +50 dBm]
I wrote a web version of the same calculator. Once you have populated all the stages, you press the button and it gives you the results. It is available on https://www.vu2ese.com/index.php/2021/05/17/cascade-calculator/.
Here is a traditional superhet built the traditional way. Let’s examine it:
-
-
- We have a band pass filter in the front-end, followed by a diode ring mixer that has about 7 db loss, the diode mixer needs a post-mix amplifier to terminate it properly,
- A 2N3904 biased at 20 mA would provide us with a strong post-mix amplifier with +30 dBm OIP3,16 dB gain and 6 dB noise figure.
- The crystal filter would have -2db loss
- A second 2N3904 as an IF amplifier with similar performance figures as the post-mix amplifier.
- The demodulator is yet another diode ring mixer with exactly the same performance as the front-end mixer.
- The audio preamp following the detector could be the common-base amplifier used for low impedance match with the diode mixer, it is traditionally biased for 0.5 mA of current, providing a good match but modest large signal capability.
You are encouraged you to visit this page(https://www.vu2ese.com/index.php/2021/05/17/cascade-calculator/) and try out your own configurations. It is a bit like the Microsoft flight simulator, you strap the wings of a Boeing 777 on a Cessna and wonder if it will fly – It is highly edifying.
“Simulation is the greater experiment” – Wes Hayward,W7ZOI
Here, as you can see, the two IF amplifiers will consume 20 mA of current each, the VFO and BFO outputs have be +7dbm (that is, 5 milliwatts) to drive the diode mixers properly. Each of those oscillators will consume 20 ma each. The buffer amplifiers that produce the 5 mW drive will also need to be biased with even higher current. I would put the total current consumption of this receiver at 100 mA.
Notice that we are measuring how the signals progress and distort each other even beyond the crystal filter. That is, consider that there are two CW signals, one is a weak 1uV signal that demodulates as a 700 Hz audio. Right next to it, about 300 Hz away is another signal that is 1mV strong (about 60 db stronger). In the receiver we are discussing, the distortion will be very heavy and weak signals will be run all over by the strong one.
On the other hand, if we use a really narrow crystal filter that will cut down the strong signal and keep it away from the stages following the crystal filter, we will have better luck snagging that DX. To simulate that, we just up the OIP3 of the stages that follow the crystal filter. The dynamic range is no longer just 67 db, we have a receiver with 93 db dynamic range!
This illustrates why some homebrew receivers will do much better operating PSK31 and FT8 where the entire band is within the 3 Khz audio bandwidth while others don’t. You have to design the radio such that all stages until the last audio amplifier are low distortion and high dynamic range.
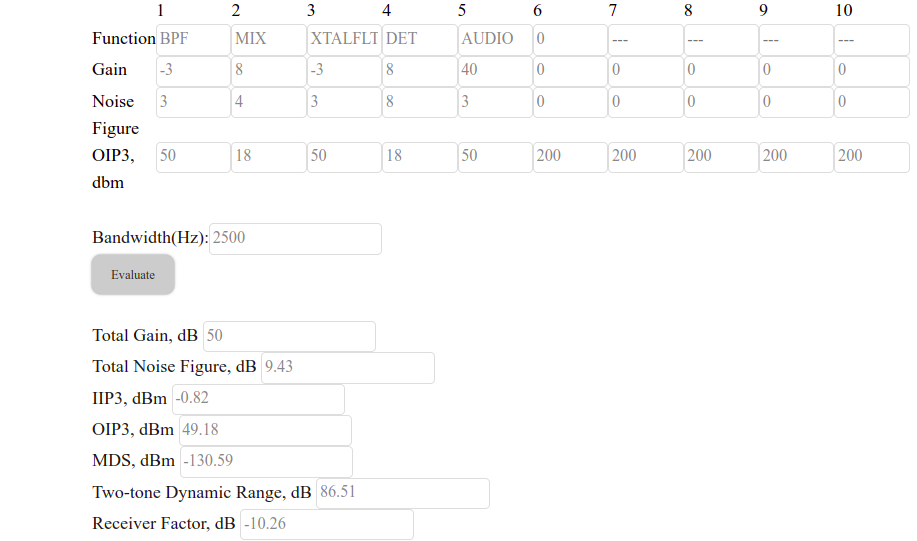
Block diagram of the Daylight Radio
Let’s now look at our proposed block diagram above of the Daylight radio. Given that the cascode mixer has 10 dB of gain and it doesn’t need post-mix amplifiers, we can eliminate the post-mix as well as the IF amplifier. The receiver consists of just three active elements : the front-end RF mixer, the detector and the audio amplifier.
I have chosen 8 db of gain instead of 10 db, this is because we will be using lower input and output impedances of 1000 ohms instead of the optimal 2000 ohms as we discussed earlier. This is to simplify some other design considerations. This receiver is providing a whopping 80 db of in-channel dynamic range. You could have your neighbour down the road with his IC7300 blasting a 10 mv signal right inside your crystal filter. If your ears survive it, you will still hear the weak 1uV signal behind it all the same. If we want to see how well it does with signals outside the crystal filter’s passband, we just increase the detector’s and audio amp’s OIP3 to +200 dBm and get a whopping 96 dB of dynamic range. This is an incredibly good figure. Ordinarily, it costs you at least 2000 dollars to buy this performance off-the-shelf. And the good news is not over yet. Each of these two cascode mixers will draw just 4 mA of power!
You will note that we also assumed that the audio has an OIP 3 of +50 dBm. How is that possible? This is because we are no longer using the conventional common base NPN transistor as the audio pre-amp. This would overload quickly. Given that the cascode mixers have a high output impedance of around 2000 ohms, we can directly drive an ultra-low distortion,, ultra-linear operational amplifier that overcomes distortion by using very high gain with feedback. That is the good ol’ NE5532.
Why the 5 MHz IF crystal filter changes everything
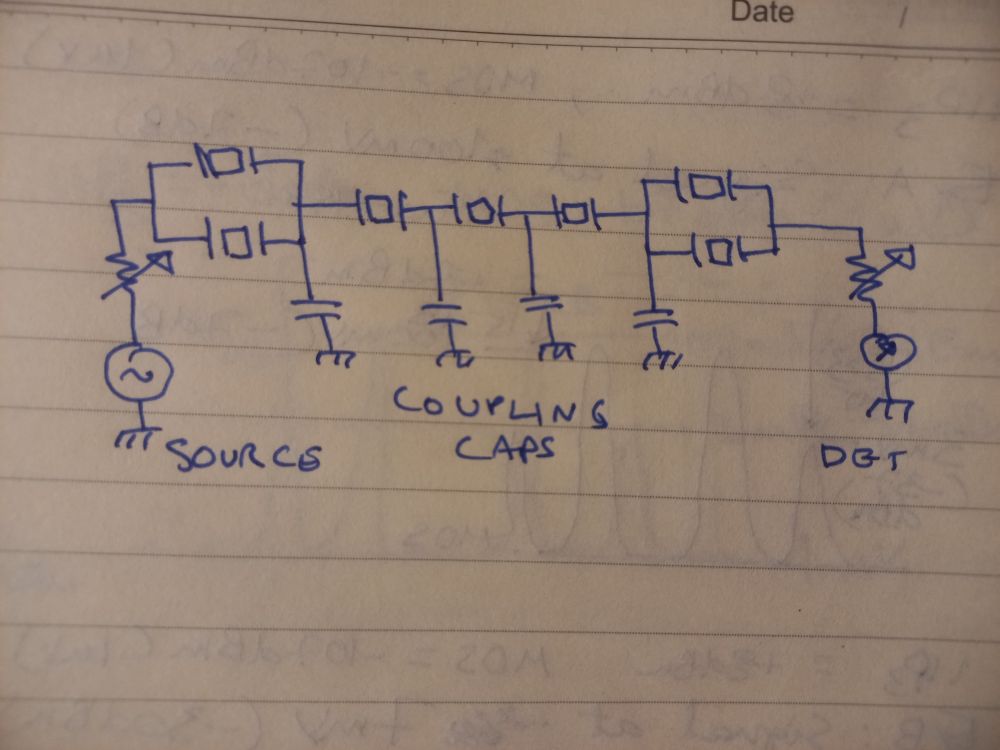
QER crystal filter
I have often based my own radios with 12 MHz or 10 MHz IF. Typically a 4 MHz or 3 MHz VFO was required to mix this IF to 7 MHz or 14 MHz. The IF choice is pretty critical. You can end up with bad birdies. Any harmonic of the VFO can mix with some harmonic of the BFO and scream at you as you tune past that point.
We chose a 5 MHz IF for three reasons:
We could build an SSB or a CW filter at 5 MHz easily. At higher frequencies, the losses with narrower filters are much more. This has to do with the Q of the filter. We will discuss this later in this paper.
At 5 MHz, we can use a 2 MHz VFO. With such low frequency, the VFO can be really dead stable; enough even for the digital modes.
An SSB filter at 5 MHzwill need an impedance around 1000 ohms, something that will directly match the cascode mixers on either side of the crystal filter!
This is a break from the tradition of basing all our stages at 50 ohms working impedance, which serves well all the time. Our test instruments are all designed to work at 50 ohms, our antennas work at 50 ohms, our cables work at 50 ohms. However, if we can afford to step out of this comfort zone and in a controlled way use the 1000 ohms as interconnects between a few stages, we can build a very optimal radio, very easily.
As this receiver was a rush job, we chose to quickly slap together a QER filter. These filters are the simplest of crystal filters, all the coupling capacitors are the same value, you can use as many crystals as you want. The filter will get steeper and the losses will mount.
An unintended consequence of too many crystals in a filter is that the group delays add up. Not all frequencies within the passband travel at the same speed through the quartz filter. It is as if the crystal filter is a highway; each lane travels at a different speed. The longer the highway, the greater will be the added up differences between signals travelling in different lanes. This is the sound of overly filtered radios. Those who have used these ‘special’ filters in their commercial rigs will testify to the badly sounding yet costly radios.
For us, about 5 stages are optimal for SSB and CW.
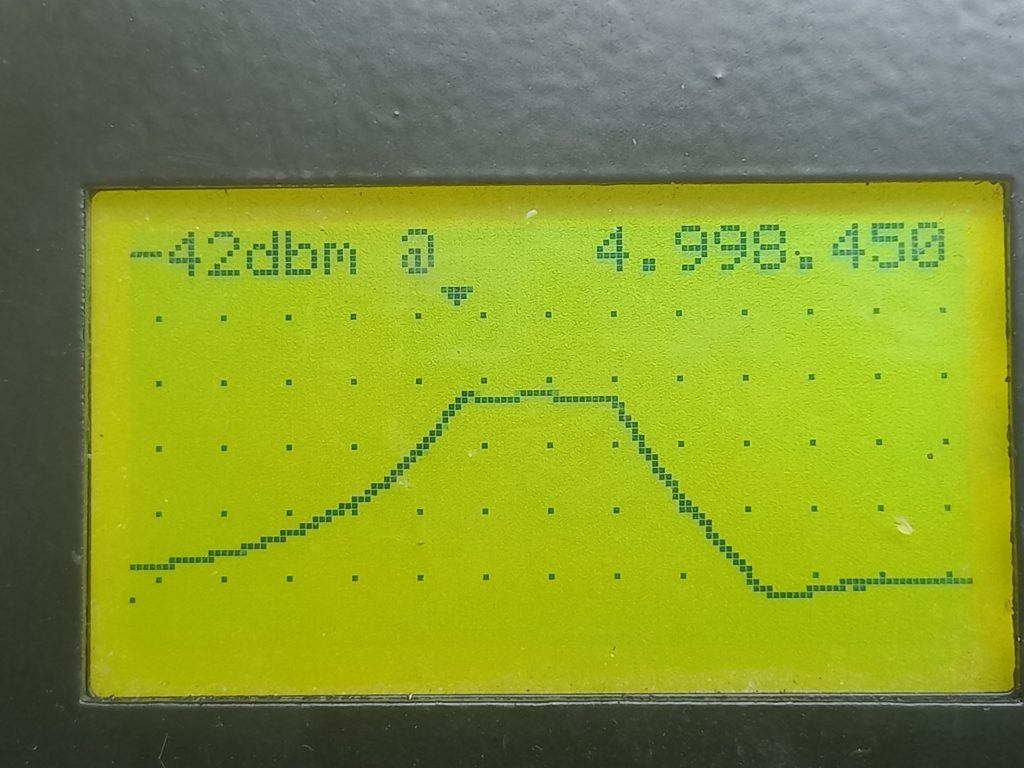
QER filter as swept on the Antuino
The QER filter can be built very simply if you have a NanoVNA or an Antuino. Please buy either of them today (Alert, I have interest in the company that produces the Antuino, further alert – the Antuino is out of stock). You start by physically building a crystal filter of the required stages with paralleled crystals at both ends. You chose a particular capacitor value, starting with 100 pF for all the coupling capacitors. Add 10 K variable resistors on both sides and sweep the filter.
To reduce the bandwidth increase the coupling capacitance, to decrease the bandwidth, decrease the capacitors. To smoothen out the ripple in the passband, increase the resistors that act to vary the termination impedance. You can experimentally arrive at the impedance and coupling capacitance for the bandwidth you desire. The variable resistance can be read off using a VOM to determine the best impedance to operate the filter at.
Here is the time for another short digression. Often, many homebrewers ask on the forums “How do I measure the impedance of my crystal filter?”. You don’t. There is no characteristic impedance of any passive filter. Most filters are, what is known as, doubly terminated. This means that filters will exhibit strange and interesting passband shapes, phase distortions, SWR etc., when they are driven and terminated by different impedances.
What we mean by the characteristic impedance of a filter is that the filter shape as advertised will happen only when it is driven and terminated at the specified impedances. A filter, by itself has no impedance. End of the digression.
The Bandpass filter
We have traditionally used double tuned circuits. It kept away the broadcaster on the image frequencies, well, mostly. There were always the stations and birdies that tuned in the wrong direction. The image suppression was just about 50 dB. Some of the even more respectable amateur radio manufacturers of American heritage have chosen to use just a doubly tuned filter in the front-end. This is a false economy.
Addition of another capacitor and inductor makes this a triple tuned circuit with far better results. You can go crazy and make a four section or even a higher section bandpass filter but the performance gains will diminish for two reasons:
-
-
- For more than 80 dB of out-of-band rejection you will need to control leakages around the filter rather than through it. You will have to box up the filter, use good cabling, shield each inductor from the other with an interstage shield, etc.
- With each stage, the losses mount. The narrower the filter, the lossier it is. It has to do with the ratio of the loaded to unloaded Q of the filters. With that in mind, here is yet another distraction on designing the band-pass filters. I have simplified it to the extreme. But here it is all the same.
Let’s start.
We have all heard of the Q factor (the Quality) for toroids, inductors, etc. Q is just the ratio of Center frequency to the bandwidth of a band pass filter. For instance, if you wanted a 100 KHz bandwidth from a 7 Mhz filter, that would be a Q of 7000/100 = 70. This is the loaded Q of the filter. As we discussed earlier, filters are specified at certain terminations. In our case, we are looking at a bandwidth of 500 KHz,
So, the QL = 14 (7000/500). (Eq 1)
What makes the Q high or low? It is the losses in a tuned circuit. Losses can be approximated by a parallel resistance too. This is where the impedance comes into play. Higher the impedance of the resonator in a filter, narrower the bandwidth. There is a simple formula to calculate that :
Rp = X * Q [ here X is the reactance of the inductor]
Let’s assume that we will use a 2 uH inductor, its’ reactance will be
X = 6.28 * Frequency * Inductance = 87.92 ohms [or more accurately +j87.92]
(We calculate reactance as an imaginary number, not important in this case)
Rp = X * Q = 87.92 x 14 = 1232 ohms.
This must be the impedance of each of our resonators. To resonate this at 7.1 MHz, we will need 250 pF capacitors in parallel to the inductor.
Next, we choose coupling capacitors. They are given as:
C = K x (Co / QL)
Here Co is the 250 pF capacitor used to resonate the inductor, K is a magic number. Different types of bandpass filters will provide different numbers, they relate to the polynomials the filters describe. Just trust me and use 0.7 here.
C = 0.7 x (250pf /14) = 12.5 pF
Instead, we will just use 10 pF.
There is a subtle detail now, the coupling capacitors add to the total capacitance seen by the inductors decreasing their effective frequency of resonance, we will need to reduce the 250 pf parallel capacitance by 25 pF to bring the resonance back to 7.1 MHz. So, each parallel capacitance is now 220 pF, this is excellent as we can buy 220 pF capacitors.
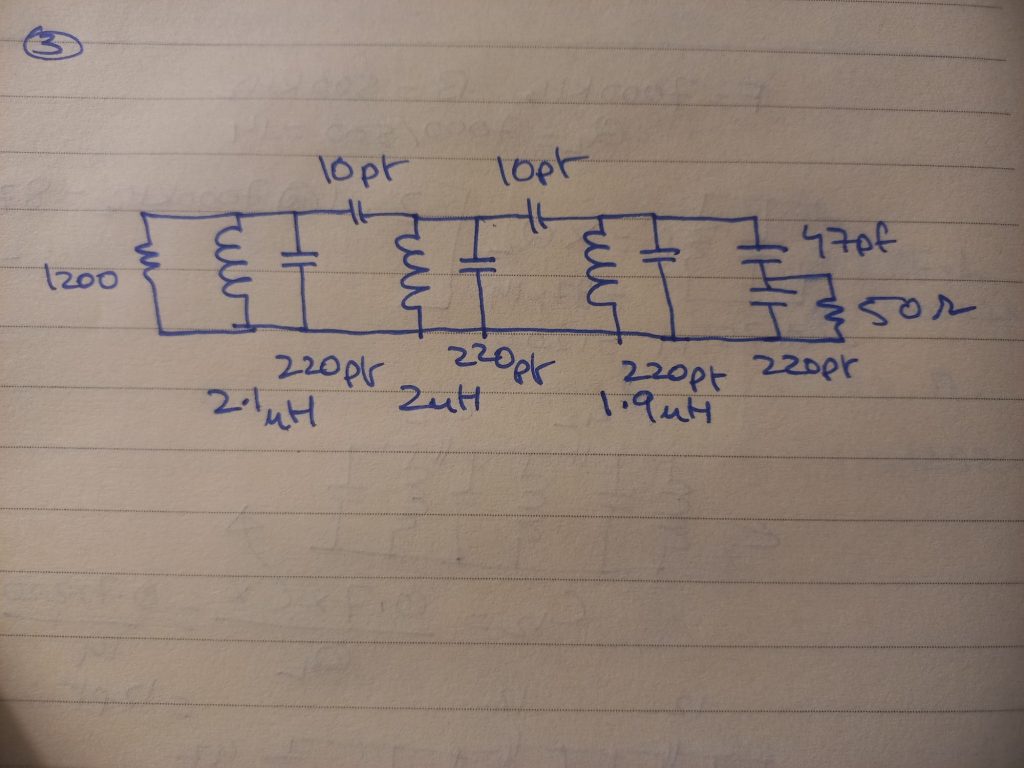
Bandpass filter, Designed.
Don’t reach for those disc ceramic capacitors yet. They are horribly lossy. Buy some decent NP0 capacitors, they are pretty cheap from the Mouser. If you buy a few hundred of them in 470 pF, 100pF and 10pF values, they will last you a lifetime of homebrewing on HF. If you are rich, order some 2.2pf as well. Remember NP0 or C0G only. Alternatively, if you can buy the polystyrene capacitors they are the best for the VFOs as well as bandpass and low pass filters. You can synthesize other values by just paralleling them up.
The bandpass filter will attach to the cascode mixer at one end that terminates in 1.2 K ohms (Actually I used 1K resistor). So, the inductor value will have to be adjusted again to resonate back to 7.1 MHz. The bandpass filter has to connect to the antenna at the other end at 50 ohms, we just have a capacitive voltage divider. The ratio of the 1000 ohms of the band pass filter to 50 ohms is 20:1. This would need a voltage division of Sqrt(20) = 4.4. Thus a 47 pf in series with a 220 pf would divide down the voltage. We will have to change the last inductor to again bring the central frequency back to 7.1 MHz.
It is simply easier to change the inductor by varying the number of turns and keeping the capacitance constant. We don’t have to physically keep winding and unwinding the inductors. We can do it in LTSpice while watching the band pass shape or we can do it in GPLA, a program that comes with the EMRFD CD. Here is my tuned version of the triple tuned circuit with the inductor values needed.
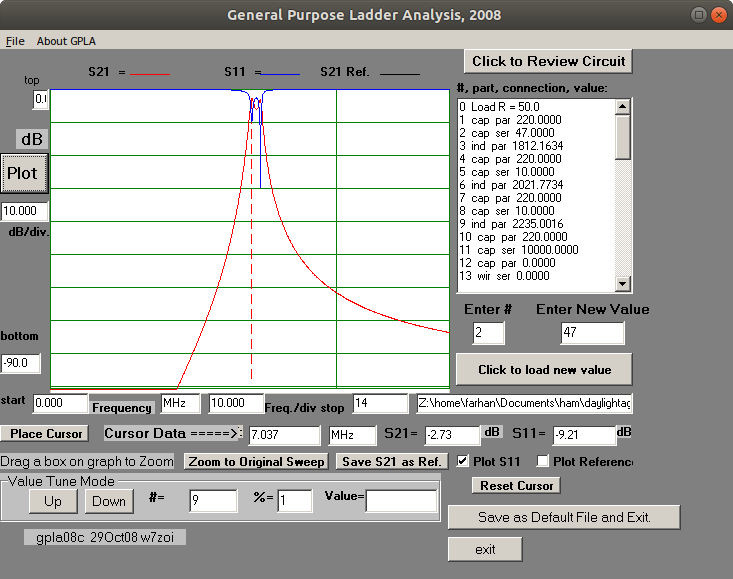
Bandpass filter simulated on GPLA
These tools are amazingly accurate in predicting the exact values. But how do we arrive at the exact values? We will have to use the Antuino or the NanoVNA. We build a simple jig that has RF connectors on two ends directly connected with a wire. We sweep this and see that there is no loss to begin with. Then we add a series trap of a known capacitor, say 220 pF and the unknown inductor and sweep it. It gives a deep notch at the resonating frequency. You know the frequency, you know the capacitance, now you can back calculate the inductance, very accurately. For instance, I needed a 2.1 uH inductor, with 220 pF this should give a notch at 7.4 MHz. In the first try with 40 turns the notch turned up at 7 MHz, I hit 7.4 MHz (after taking off 8 turns,) at 33 turns.
3D printed toroids
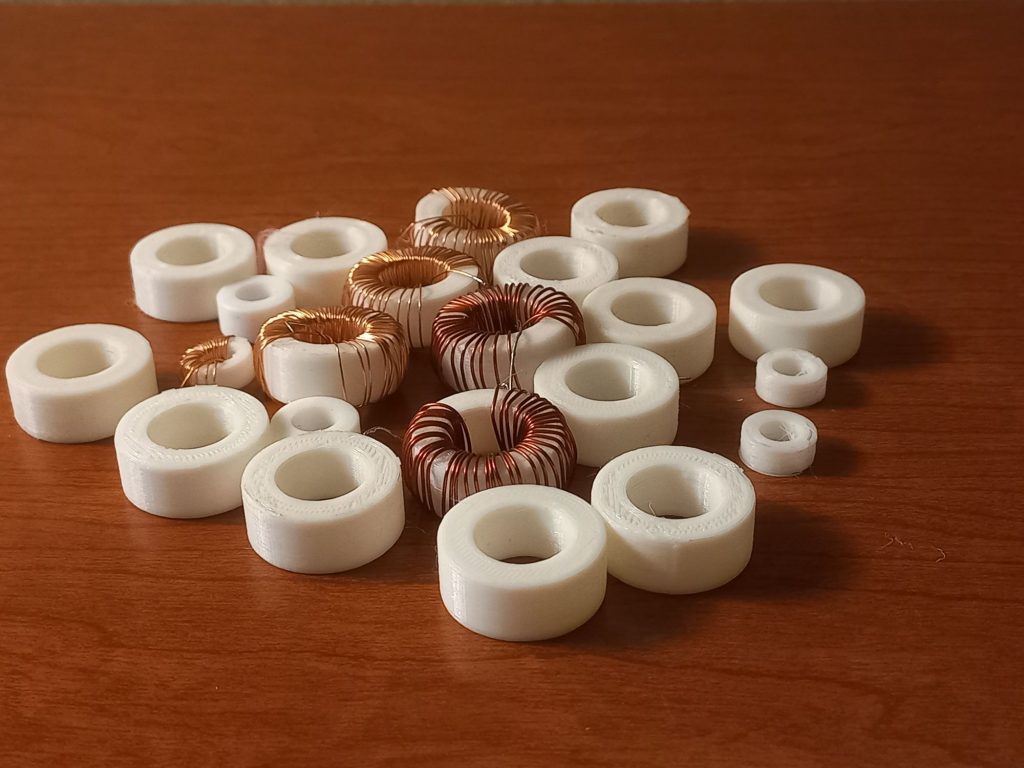
Yet another digression, this one will save you money … You can 3D print toroids!! We experimented with various sizes and finally landed up with 1 inch outer dimension toroids that reports a Q of about 150. Pretty reasonable for toroids that are almost free. You can print 10 of them at a time on the a home 3D printer. This was a big learning from this project.
We have used them in the bandpass filter as well as the low pass filter. They perform pretty well. To calculate the inductance, you have to square the number of turns and multiply with two.
Inductance [in nano henry] = 2 * (number of turns)2
The Receiver, then
Here is the receiver part of the radio in all its glory.
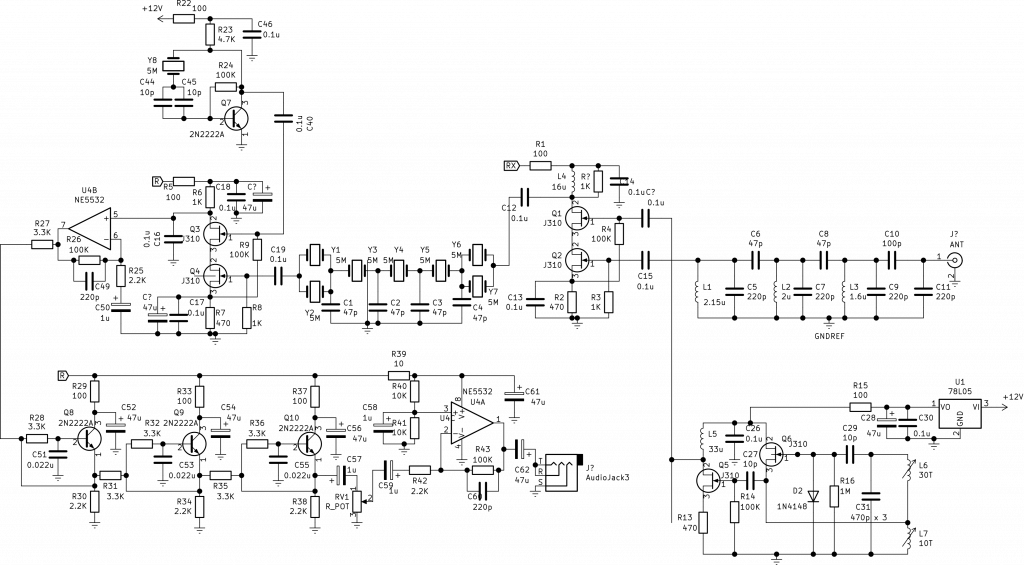
Receiver Portion. Click on it for a larger version
The Audio filter
The active audio filter is a surprise. This is really a worthwhile addition. With less than 1 dollar worth parts, the receiver audio improved immensely. Instead of using op-amps, we just use 2N2222A as unity gain amplifiers, this is just to demonstrate that there are many ways to get there.
The audio filter has a cut-off around 2 KHz. SSB and CW, both sound warm and nice. The difference with and without the audio filter is dramatic, you want to put it back on again immediately if you throw the switch to bypass it. Note that the entire audio chain from the demodulator to the volume control is DC coupled. This needs the cascode demodulator’s audio output to have a DC operating voltage of around 8 volts so that there is enough head room for the last of the filter transistors to not bottom out at large signal levels. The audio cut-off is determined by the 3.3K resistance. I misplaced them in the shack and soldered 1K and 2.2K resistors in series. For CW work, you can reduce it to 1K.
The PTO
The VFO is another 3D printed part. Instead of hard to find variable capacitors, we use a variable inductor to tune the band. After all this is 2022. Slow motion drives are hard to find. They are messy to install, they exhibit backlash. Instead, we used a simple 3D printed PTO. It is essentially an air inductor about 5 cm long that can be horizontally mounted on the PCB with four M3 screws. It has two slots where you can insert nuts for 1/4-20 screws. A nice long brass 1/4-20 bolt is the main tuning element that moves in and out, guided by the two nuts held firmly in their slots. It provides around a decent, 25 KHz tuning per turn. It took 40 turns of 22 swg enamelled wire to fill the former end to end. The tap is at 10 turns. The 3D printed PTO is really easy to build. It prints in 15 minutes. It is dead stable at 2 MHz. To net to the exact frequency range, you can add or remove turns on the PTO former.
Three polystyrene capacitors of 470 pF each, in parallel, brought the tuning in the range of 2 MHz. A serendipitous advantage of 2 MHz PTO that tunes upwards is that a regular frequency counter can be used for accurate tuning.
A 78L09 was used as the PTO’s voltage regulator. A simpler choice would have been to use a 9.1v Zener diode. We didn’t. The zener regulator works by sinking current that the VFO isn’ consuming. This current converts to heat which can drift the VFO. On the other hand, if we reduced the current, the Zener would starve and turns noisy and the regulation would suffer too. Instead, the free running VFOs are best powered by 78L09. They hardly sink any current, they are noise free if they are properly bypassed both at the input and the output. For a VFO you must also bypass it at audio to remove any hum modulation. A 1uf electrolytic capacitor mounted very close to the terminal output pin is recommended.
The PTO has the minor visual disturbance of a knob that comes out and goes back in. It is best to leave it tightened against the chassis when not in use or that take it away with you to prevent other prying hams from using the radio!
We use a low power single JFET as a buffer. The cascode mixer and the transmit mixer hardly need any driving power and hence the bias current is kept low.
The PTO is built with just the component leads themselves. A box made from thin copper sheeting is soldered around it to keep it thermally stable and prevent the RF from the transmitter, etc from coupling into the PTO. Without the shielding, there was a transmit spur at 8 MHz, probably from the PTO’s 4th harmonic. It went away with the shielding and it was never fully investigated.
The Transmitter
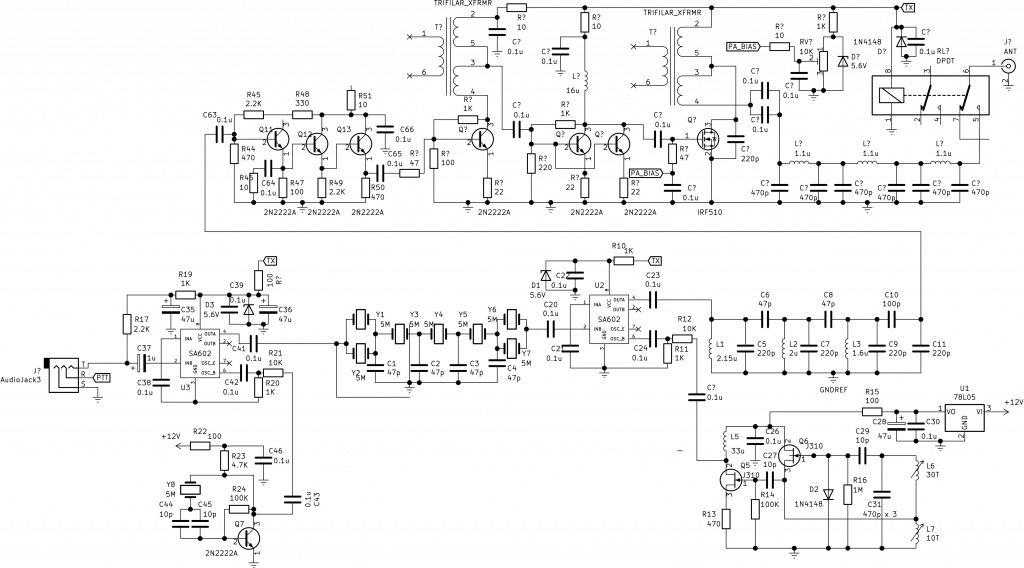
Click on the image for a larger picture
We could have used separate transmit and receiver filters and only used the PTO and the BFO as the common elements. This has been the usual practice except for the BITX line of radios.
A future plan to make the radio have full break-in will need a rebuild with a separate set of filters. To save time and space, we switched the filters between the receive and transmit sections.
The transmitter uses NE612 (also sold as SA602) as the modulator as well as the transmit mixer. Isn’t this a bit of a bummer. After all, isn’t NE612 thought to be an underperformer best used in boy scout direct-conversion receivers?
The NE612 can’t match a diode mixer in a receiver application, but it has its own advantages in a transmitter. This is where science takes over the lore.
The NE612 is a doubly balanced mixer with very good suppression of the injected oscillator as well as the input signal. It is hard to surpass it as a DSB modulator. It has an output intercept of -10 dBm, This would mean that if we extract a -20 dBm signal from it, then the IMD will be 30 dB down. Respectable level for the IMD is -26 dB below the peak tones. A -20 dBm signal is a strong 30 mV signal with IMD down by -30 dBc. You will just have to amplify it up.
Additionally, the NE612 mixer input is sensitive enough to not need a microphone amplifier. A termination insensitive amplifier built with three transistors provides a 20 dB boost to produce a 0 dBM signal that drives a traditional amplifier made from 2N2222As and an IRF510. We used similar 3D printed toroids in the low pass filter as well.
At first, a two section output low pass filter was tried. The 14 MHz harmonic was just below -43 dBC, another section brought it below the 50 db level. Now, the output harmonics are virtually unnoticeable.
The T/R switching can be improved. An electronic T/R switch can be made that can be the the start of a full-break CW modification for the radio.
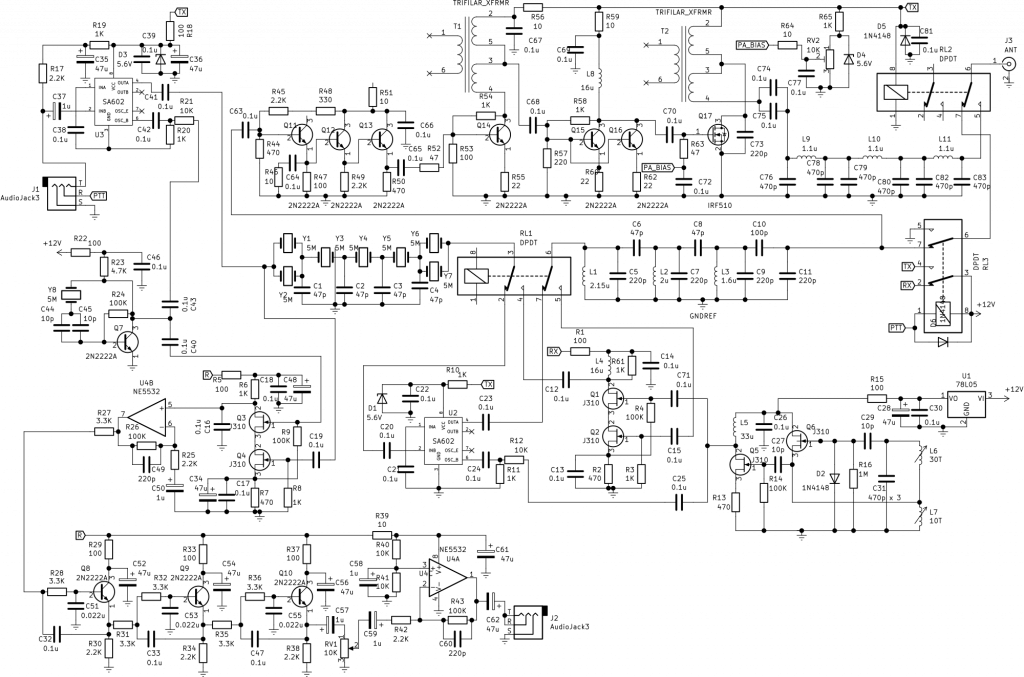
Full circuit (click to enlarge)
Concluding notes
There are some surprises here. Except for the transmit power chain, no broadband transformers were used anywhere in the design. This was an unstated design goal. They can be easily removed from the PA as well. An L network can easily substitute them. We will still need ferrites for the RF chokes.
We added a frequency counter bought off the net for five dollars as a read-out. The receiver squeals each time it is connected due to the horrible RFI generated by the counter. Until a low noise frequency counter is homebrewed, we have an ‘arrangement’. There is a push button that switches on the frequency counter for you to ‘spot’ yourself. It is a little like the frequency markers of days gone by. Press the button to know where you are.
The receiver is really pleasing. I personally have an almost 99:1 ratio of receiver to transmit. I listen to bands almost daily, for hours while working. Receiver audio quality is important for me. The Daylight radio doesn’t wear you out. The active filter, very low phase noise PTO, broad crystal filter, make it a pleasure to use.
The few contacts were interesting. We are able to regularly decode FT8. I am not sure if this is a happy accident or repeatable performance. We are yet to complete a two way FT8 QSO. Audio transmission is reported to be clear and intelligible.
The Daylight Again radio is also an ode to the darkness of the last two years. To celebrate us who are gathered here again, to invoke the soldersmoke and our endeavours to get across to each other, entirely on our own.
I owe a great deal to my fellow hams at Lamakaan Amateur Radio Club for their help. Radhakrishna “RK”, VU2EHR designed the 3D models of the toroids and the PTO which form the basis of this work
Venu, VU2BVB and Anil, VU2DXA helped with testing and making the lunchbox chassis. A big thank you to Wes, W7ZOI who wrote the seminal works that form the science of all that we do. His tool, Cascade, is central to planning any radio, analog or not.
Much of the filter theory too is distilled from a close reading of the book Experimental Methods in RF Design. I have heard that the book will not be printed anymore. That’s tragic. All important texts should not be viewed primarily as commercial ventures. I hope some of us can impress upon the ARRL to continue to print it or release it out as a free/paid PDF.
Leave a Reply